Latest on endocrinology, cardiology, pharmacology
Amy Carpenter Aquino
July 2023—The human microbiome has been called the forgotten organ, and at one time it was. But not in the past 10 years.
James Versalovic, MD, PhD, pathologist-in-chief and chair of pathology at Texas Children’s Hospital, made that clear in his talk at the Association for Molecular Pathology meeting last year.
For the human microbiome, “the past decade is akin to the 1990s and human genome research,” he said, “where we had to lay the road map. We had to construct the cartography of the human microbiome,” which began formally with the National Institutes of Health in 2007 but took root in 2012 with seminal publications on the NIH-supported Human Microbiome Project.
Since then, he said, “it’s been quite a ride.”
“I’m firmly convinced that in this decade and the next we will see the same amount of progress that we saw with human genome research in the last 20 years,” said Dr. Versalovic, the Milton J. Finegold professor and vice chair of pathology and immunology at Baylor College of Medicine. And it’s about more than genes. “It’s about cells,” he said. “It’s about communities of cells, similar to any organ in the human body.”
Dr. Versalovic is director of the Texas Children’s Microbiome Center. “Over the last 10 to 15 years,” he said, “we’ve discovered differences in metabolic pathways and the human gut microbiome in children versus adults.” Gut microbes in children may be producing more B-complex vitamins and folate. “Those pathways are enriched in children, and it may be that children with certain metabolic disorders have deficiencies in these pathways.” They’ve identified genes and pathways that could be used to address and correct deficiencies via oral supplementation with B-complex vitamins, for example (Hollister EB, et al. Microbiome. 2015;3:36).
Correlations are being established between the gut microbiome and human blood biochemistry, Dr. Versalovic said. “You’re going to be seeing more and more fusion of the two because many of the metabolites in human serum and plasma are derived from microbes in the gut and other body sites.”
Dr. Versalovic hit the highlights of microbiome research as they relate to endocrinology, cardiology, and pharmacology, beginning with microbial modulation of insulin resistance and type 2 diabetes.
“Branched-chain amino acids—leucine, isoleucine, valine—are important in human metabolism,” he said (Fig. 1). These amino acids then feed the tricarboxylic acid (TCA) cycle, and oxidation of the amino acids can be implicated in different genetic disorders (Vanweert F, et al. Nutr Diabetes. 2022;12[1]:35).
This was brought to light, he said, with a study of 277 non-diabetic Danish adults for whom gut microbial data were available (Pedersen H, et al. Nature. 2016;535[7612]:376–381). The authors assessed the role of the gut microbiome as a source for key features of the serum metabolome profile, predicting metabolic and cardiovascular disorders in non-diabetic lean and obese people. They found these individuals to have elevated branched-chain amino acids that correlated with a state of insulin resistance and prediabetes, Dr. Versalovic said. “They also found that key organisms, namely Prevotella copri and Bacteroides vulgatus, correlated with elevations in branched-chain amino acids in human serum or plasma.”
P. copri and B. vulgatus are also the genes that encode for biosynthesis in branched-chain amino acids contributing to the metabolite pool, he said. Other microbes that may have contributed to elimination of branched-chain amino acids via specific transporters were deficient in the gut microbiome of insulin-resistant individuals. “So we begin to see the correlation between changes in the microbiome and potentially new biomarkers, such as specific amino acids that may augment known analytes such as hemoglobin A1c and serum blood glucose. In turn, new combinations of old and new biomarkers could improve the stratification of patients with insulin resistance, prediabetes, and ultimately type 2 diabetes,” Dr. Versalovic said. These findings may point in time to improved diagnostic testing and disease monitoring strategies for patients treated for type 2 diabetes.
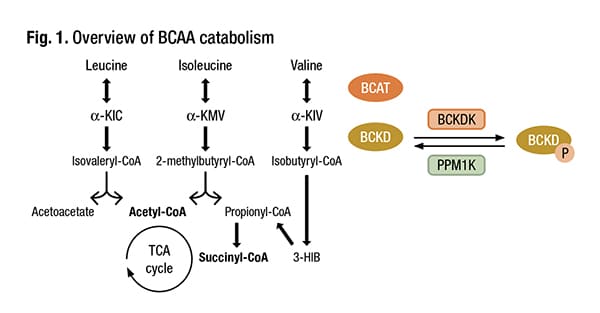
Schematic overview of BCAA catabolism. BCAT branched-chain amino acid transaminase, BCKD branched-chain keto acid dehydrogenase, α-KIC α-ketoisocaproate, α-KMV α-keto-methylvalerate, α-KIV α-ketoisovalerate, 3-HIB 3-hydroxyisobutyrate, BCKDK BCKDK kinase, PPM1K, BCKDK phosphatase. Adapted from Neinast et al.* Credit: Vanweert F, et al. Nutr Diabetes. 2022;12[1]:35. CC BY 4.0 License- https://creativecommons.org/licenses/by/4.0/ *Neinast M, et al. Annu Rev Physiol. 2019;81:139–164.
These connections in the biology are now being drawn, he said, noting that follow-up studies documented elevations in branched-chain amino acids in patients with type 2 diabetes. “And BCAA elevations correlate directly with the levels of hemoglobin A1c” (Medina-Vera I, et al. Diabetes Metab. 2019;45[2]:122–131). This raises the question: Can branched-chain amino acids be used to monitor disease in addition to HbA1c?
“There’s a real argument now for possibly adding measurements of branched-chain amino acids by mass spectrometry in the clinical laboratory to refine the stratification, assessment, and monitoring of patients who have or are at risk for type 2 diabetes,” Dr. Versalovic said. And it’s microbiome science that highlighted these findings. “What we’re learning about the microbiome is emphasizing potential pathways that may be important for diagnosis, monitoring, and ultimately treatment and prevention of different chronic disease states,” he said.
Intervention strategies to increase branched-chain amino acid oxidation and/or lower BCAA levels are important to investigate as a potential treatment for metabolic diseases, Vanweert, et al., write in their review (Vanweert F, et al. Nutr Diabetes. 2022;12[1]:35). There are key pathways, Dr. Versalovic said, in the oxidation of BCAAs in the human mitochondria. “And the rate-limiting step is the enzyme BCKDK,” or branched-chain ketoacid dehydrogenase kinase, which “can be blocked by particular pharmacologic inhibitors.” He cites as an example sodium phenylbutyrate, which has been used to treat patients who may have certain urea cycle disorders and maple syrup urine disease. The fibrates used in combination with statins to prevent cardiovascular disease also block this pathway. “With these drugs, we may be able to block BCKDK, the rate-limiting step, and that would result in decreased plasma branched-chain amino acid concentrations.”
“Ultimately, the effects on branched-chain amino acids may have important implications for the mTOR pathway and insulin resistance,” he said, noting that it’s an emerging field with in vitro and in vivo animal model data as well as human data.
On the topic of microbial modulation of cardiovascular disease, Dr. Versalovic cites the work of Stanley Hazen, MD, PhD, and colleagues of the Cleveland Clinic Lerner Research Institute on trimethylamine (TMA) and trimethylamine N-oxide (TMAO). In a review article, Brown and Hazen write that the TMAO pathway “is a meta-organismal metabolic pathway whereby nutrients that are present in high fat foods . . . can be metabolized by several distinct gut microbial enzyme complexes (CutC/D, CntA/B, YeaW/X) to generate the primary gut microbial metabolite TMA” (Brown JM, et al. Nat Rev Microbiol. 2018;16[3]:171–181). Other reviews, they write, highlight the clinical relevance and therapeutic potential of the TMAO pathway in cardiovascular disease.
Red meat, eggs, saltwater crustaceans and fish, and dairy products have relatively high amounts of choline and L-carnitine that can be converted by bacteria in the gut through well-defined biochemical pathways, Dr. Versalovic said. CutC/D is the enzyme that converts choline to TMA. A separate pathway, CntA, converts L-carnitine to TMA. TMA is then metabolized in the liver by flavin monooxygenases to TMAO. “These findings appear to be clearly relevant” and are yielding a new biomarker (or new biomarkers) for human cardiovascular disease, Dr. Versalovic said. Dr. Hazen’s group and others have now fully documented the connection, he said, between increases in TMAO and elevated risk for atherosclerosis, plaque development in blood cells, heart failure, increase in foam cell macrophage formation due to upregulation of scavenger receptors, and increase in platelet aggregation and hyperreactivity.
TMAO is licensed and used as a clinical test to evaluate this additional biomarker for measuring cardiovascular disease risk, and there are preclinical trials to inhibit TMAO pathways in the microbiome to reduce the risk of CVD, Dr. Versalovic said.
In their 2021 review of how gut microbiota contributes to heart failure, Zhang, et al., write that TMAO “is closely associated with the poor survival of HF patients, which helps clinicians to screen out high-risk groups and pay more attention to their follow-up monitoring as well as enhance HF-related treatments.” The problem, they add, is the lack of diversity in the existing clinical research subjects, who are mainly Caucasian, and additional cohort studies enrolling patients of other races are needed (Zhang Y, et al. Transl Res. 2021;228:109–125).
Drug discovery and development are ongoing, with the aim of inhibiting TMAO “based on this new paradigm for atherosclerosis and relevance to type 2 diabetes,” Dr. Versalovic said. TMA lyase is the enzyme most commonly found in the microbiome that is linked to TMA production. It is present in a variety of bacterial species that lead to conversion of dietary choline to TMA (Steinke I, et al. Front Physiol. 2020;11:567899).
Dimethyl-1-butanol (DMB), a compound found in red wine and in higher amounts in balsamic vinegar and grapeseed and olive oils, “is a powerful inhibitor of the bacterial TMA lyase in the gut microbiome,” Dr. Versalovic said. DMB and other derivative compounds are being developed to target the CutC/D pathway in the microbiome to try to reduce cardiometabolic disease risk long term (Wang Z, et al. Cell. 2015;163[7]:1585–1595).
Berberine, a plant alkaloid found in some food products and supplements, has been documented to block the conversion of choline to TMA (Ma SR, et al. Signal Transduct Target Ther. 2022;7:207).
The human microbiome over a lifetime is exposed to thousands of xenobiotics—any substance or chemical compound that is foreign to the body or ecosystem, such as pesticides, printing ink, food additives, and drugs. “Think of residual plastic in your water,” Dr. Versalovic said.
While antimicrobial agents are most commonly associated with an impact on the microbiome, the focus in his talk was drugs for which it was unclear, until recently, whether there was an impact on or by the microbiome. Corticosteroid metabolism by human gut microbes, specifically Clostridium scindens, can convert glucocorticoids into androgens. Human microbes will convert C-21 corticosteroids to androgens and other metabolites (Ridlon JM, et al. J Lipid Res. 2013;54[9]:2437–2449). “This may be relevant to patients who are medicated for chronic autoimmune and other conditions that require the use of corticosteroids over time,” he said.
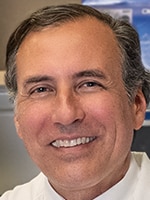
Dr. Versalovic
“We’re now beginning to understand the different metabolic pathways in the human microbiome that may affect drug metabolism.”
Since human microbes can modify drugs and medications through various biochemical pathways, such as acetylation and β-glucuronidation, leading to activation or inactivation of drugs or toxic metabolite production, the implications for pharmacology and patients are important. “I could see a future where we’re examining both bacterial genes and human genes and assessing whether drugs are going to work in different sets of patients,” Dr. Versalovic said. “And we as a pathology community are going to have to be front and center in helping our clinical colleagues navigate this very complex genetic landscape.” The number of genes in the human genome is far lower than the 2 million in the human microbiome. “One hundred-fold more bacterial genes may be residing within you.”
“This is a massive genetic and biochemical microbial landscape in the human body,” he said, “and we’re obviously going to need high-throughput, latest-generation sequencing technologies and mass spectrometry to be able to discern signal from noise.” He’s optimistic: “This is a manageable problem and health care challenge for the next decade.” Pathologists can help lead the way, he said, in providing new diagnostic and patient-monitoring strategies based on a “holistic world view of human pathology and the microbiome.”
Amy Carpenter Aquino is CAP TODAY senior editor.