William Check, PhD
September 2014—At the 30th Annual Clinical Virology Symposium this spring, Gregory Storch, MD, related a typical case of a febrile child seen in the emergency department. Dr. Storch, a professor of pediatrics at Washington University School of Medicine, described a 20-month-old boy with a fever of 40°C, rash, cough, and nasal congestion but no gastrointestinal symptoms. White blood cell count was 7,800/µL. Blood culture was negative and a chest x-ray showed mild peribranchial thickening. Diagnosis, Dr. Storch says, was “viral syndrome.” The patient got a dose of ceftriaxone, which was “reasonable,” in Dr. Storch’s view, in light of the patient’s fever and the presence of bands on the peripheral blood smear.
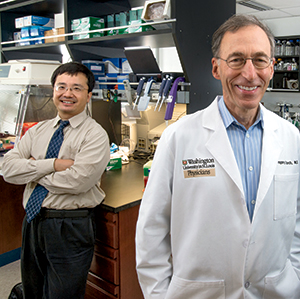
To define viruses in children with fever without cause, Dr. Gregory Storch (right) and colleagues conducted the Febrile Children Study. Richard (Xinran) Hu, MD, MPH (left), played a key role in studies on human gene expression profiles.
This case typifies “fever without a source,” a common pediatric problem in physicians’ offices and in the ED, said Dr. Storch, who is also director of the Division of Pediatric Infectious Diseases and of the Division of Pediatric Laboratory Medicine at St. Louis Children’s Hospital. Many of these children receive antibiotics because “bacterial infection is always a serious concern,” he noted.
In his CVS talk, Dr. Storch spoke about his group’s efforts to improve the targeting of antibiotics. He posed the question: Can we do better using advanced molecular techniques? His work has foc-used on two newer techniques: next-generation sequencing—genomics, and gene expression profiles—transcriptomics. “This is where we have to think about moving in the future,” Dr. Storch tells CAP TODAY.
Of the possibility of translating the group’s results with transcriptomics into clinical utility, Dr. Storch says: “We can’t do that tomorrow, but it is not that far into the future. If we approached a specific clinical syndrome, we could go tomorrow and do a clinical study in that patient group using some of these markers. It would require more work to transfer [this technology] to samples other than blood or to other clinical syndromes, but the path is definitely open for people to do that.” Dr. Storch expects to see this method become part of routine clinical testing in perhaps five years.
He cites prior work with gene expression profiling in acute infections by a group led by Octavio Ramilo, MD, head of pediatric infectious diseases at Nationwide Children’s Hospital in Columbus, Ohio (Ramilo O, et al. Blood. 2007;109:2066–2077). Using what they call “discriminative transcriptional signatures,” Dr. Ramilo and his colleagues achieved 90 percent to 95 percent correct classification. They concluded that “[M]icroarray analyses of patient peripheral blood leukocytes might assist in the differential diagnosis of infectious diseases.”
A group at Duke University School of Medicine is working, too, with gene expression profiles as advanced diagnostics for distinguishing viral from bacterial infections. “The major driving issue is emergence of antimicrobial resistance,” says Christopher Woods, MD, MPH, who notes it’s a global problem owing to inappropriate antibiotic use.
“When someone presents with a possible res-piratory infection, it is the same in Nepal or Sri Lanka or Africa or downtown Durham. Our ability to determine whether a virus is causing their symptoms or a bacterium is extremely limited. One cannot usefully do it with clinical symptoms or current laboratory tests,” says Dr. Woods, a professor of medicine and of pathology at Duke and chief of the Infectious Diseases Division at the Durham VA Medical Center.
“What we have spent most of the last decade doing,” he tells CAP TODAY, “is working to develop a diagnostic test that provides a clinician with a robust result and an accurate result that distinguishes whether the patient has a bacterial or viral infection and that would drive therapeutic intervention.”
David R. Hillyard, MD, director of molecular infectious disease testing at ARUP Laboratories, is familiar with the work of both groups. He arranged for Dr. Storch to speak at the 2013 meeting of the Association for Molecular Pathology and for Dr. Woods to speak at this year’s AMP meeting in November.
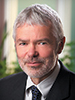
Dr. Hillyard
“There is a tremendous potential and a need that has been longstanding and long discussed,” Dr. Hillyard says. Data presented so far from various groups suggest a good likelihood that gene expression profiling will become an important test. “We had seen in the general testing space a very rapid transition during the past several years from insensitive antigen detection or slow, relatively insensitive culture methods to monoplex molecular tests and now to multiplex molecular tests,” says Dr. Hillyard, who is also a professor of pathology at the University of Utah. “Some will be point-of-care or near-point-of-care tests very soon,” he adds.
But there is a big difference between detecting a pathogen with any of these methods and detecting the pathogen that is causing the disease process, Dr. Hillyard says. “Availability of very sensitive multiplex methods and methods detecting in some cases multiple pathogens really increases the pressure for rapid and rational clinical decisionmaking to define, maybe by host response, whether you have just the presence of a pathogen or have disease caused by that pathogen.
“These methods are gaining traction. With groups going full speed, they will likely be reduced to practical tests in the near future. We will see,” he says cautiously. “But this approach has a good chance of being very impactful.”
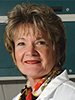
Dr. Ginocchio
Christine C. Ginocchio, PhD, MT(ASCP), a professor at New York’s Hofstra North Shore-LIJ School of Medicine and vice president of microbiology affairs at BioMérieux, also sees the “extremely great promise” in gene expression profiles, perhaps in combination with current biomarkers such as lactate and procalcitonin. “That work is extremely interesting, looking at gene expression profiles, looking at specific signatures to be able to differentiate viral from bacterial infection,” Dr. Ginocchio says. “There will possibly be a combination of markers.” With a variety of biomarkers, she says, “we should be able to get to the point where we can make the distinction whether we are dealing with bacterial or viral infection and help direct appropriate use of antibiotics.”
Viral infections in children became a focus of attention after the successful introduction of childhood vaccines for encapsulated bacteria, including Haemophilus influenzae type B (1985 and 1987), Streptococcus pneumoniae (2000 and 2010), and Neisseria meningitides (2005). Infections with these organisms were “virtually eliminated” in children under age five, Dr. Storch said in his CVS presentation. Now the number of children with fever without a source who actually have bacteremia is about 0.25 percent, he said. Five to 10 percent have bacterial urinary tract infections.
To define viruses present in children with fever without a source, Dr. Storch and his colleagues conducted the Febrile Children Study. To fund the work, they wrote a grant for a demonstration project in the Human Microbiome Project, or HMP. “It was a break with tradition,” Dr. Storch says. “We changed the focus of the HMP to include viruses.”
Investigators enrolled children ages two months to 36 months with fever higher than 38°C and no obvious source, as well as controls—afeb-rile children having same-day surgery. Blood and nasopharyngeal swab samples were tested with an extensive battery of virus-specific PCR assays.
Viruses were detected in 76 percent of 74 children with fever and no apparent source. Among 17 children with fever and a definite or probable bacterial infection and 116 afebrile children, the rates of virus recovery were significantly lower, 41 percent and 35 percent, respectively (Colvin JM, et al. Pediatrics. 2012;130:e1455–1462). Adenovirus, human herpesvirus 6 (HHV-6), parechovirus, enteroviruses, and bocavirus made up 64 percent of viruses identified in febrile children by PCR.
Physicians made treatment decisions without knowing the PCR results. Half of children with viral infections but no evidence of bacterial infection were treated with antibiotics, including 90 percent of adenovirus infections. As expected, “Physicians have a hard time being sure that patients have viral infections and not serious bacterial infections,” Dr. Storch says.
In the second phase of the Febrile Children Study, 176 samples were analyzed by unbiased next-generation sequencing on an Illumina GA IIx following nonspecific amplification. Dr. Storch called the decision to go ahead with NGS “a bit dicey at the time,” because of the short reads produced by then-available sequencers. “It worked out,” he said. A wide range of RNA and DNA viruses was detected from 25 viral genera. Expected pathogens were found—adenoviruses, enteroviruses—but also unusual ones of unknown pathogenicity, such as astrovirus MLB-2 (Wylie KM, et al. PLoS One. 2012;7:e27735). Samples from febrile children contained a greater number of viral sequences, as well as viruses from a broader range of genera, than did samples from afebrile children, particularly in plasma. No sequences of pathogenic viruses were found in the plasma of afebrile children.
Each method—PCR and NGS—detected viruses that the other did not. In the comparison of PCR and NGS for their ability to detect virus, Dr. Storch says, “Concordance was somewhat disappointing. In our hands, sequencing was not as sensitive as directed PCR.” It’s possible that newer NGS techniques would detect more viruses. “We did experiments where we did deeper sequencing,” Dr. Storch says. “In some instances we were able to detect viral sequences detected by PCR but not by our original sequencing technology. This technique is evolving. Our method was early days.”
In its favor, sequencing can detect many more viruses because PCR is directed. “A definite strength of sequencing is that it is much less limited in the range of viruses it can detect,” Dr. Storch says. “With PCR you can only find what you already know. And we can’t make a PCR for every virus.” One approach is the one they followed with anelloviruses (also known as Torque teno viruses): They were detected in patient samples by NGS and found to be associated with fever (McElvania TeKippe E, et al. PLoS One. 2012;7:e50937). The laboratory then made PCRs to detect and discriminate among different anelloviruses.
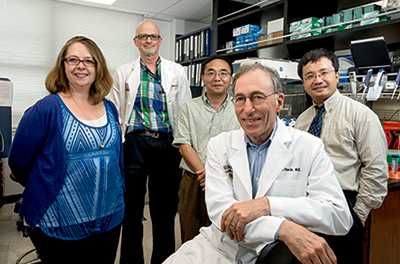
The team at Washington University (from left): Kristine Wylie, PhD, Department of Pediatrics instructor; Richard Buller, PhD, D(ABMM), Special Projects Laboratory co-director and director of the St. Louis Children’s Hospital virology lab; Jinsheng Yu, MD, PhD, instructor in genetics, Genome Technology Access Center; Dr. Storch; and Richard (Xinran) Hu, MD, MPH, Washington University Genome Institute.
In Dr. Storch’s estimate, when the laboratory is searching for a viral cause for unexplained fever, NGS could fit after screening with available PCR assays. “We are making great progress with multiplex PCR panels,” he says, noting that several respiratory panels have been cleared by the FDA, and a result is available within hours. Sequencing might be invoked when a screening panel is negative. Still, “I don’t think we are able to integrate sequencing into clinical care right now. It is a few or more years away,” he says.
In the third segment of the Febrile Children Study, Dr. Storch and his colleagues analyzed selected patient samples by gene expression profiling. They were exploring two hypotheses: first, that gene expression patterns in human WBCs can provide a fingerprint of viral infection that distinguishes it from bacterial infection, and, second, that the host/human gene expression profile in WBCs can distinguish between symptomatic (febrile) and asymptomatic (afebrile) infection. “We hope it can address the problem of multiplex assays detecting so much rhinovirus. When is it meaningful, and when is it just background carriage? Host response might distinguish those two states,” Dr. Storch says.
Samples used were from 24 subjects in the Febrile Children Study who had a single virus by PCR (11 adenovirus, eight febrile and three afebrile; 10 HHV-6, eight febrile and two afebrile; and three enterovirus), eight subjects with confirmed bacterial infection, and 22 febrile controls with no virus. Data were displayed as heat maps of expression patterns on microarrays and Venn diagrams of probes associated with each type of patient (Hu X, et al. Proc Natl Acad Sci USA. 2013;110:12792–12797).
In general, Dr. Storch says, “when you’re infected with adenovirus or HHV-6 your body goes crazy.” Changes in regulation were seen in more than 5,600 host genes. For HHV-6, 3,500 genes had changed expression, and infection with enteroviruses up- or downregulated 4,200 genes. Bacterial infections did not change the gene expression profile as dramatically as did viral infection.
Several statistical programs were used to create expression profiles that distinguished between conditions (for example, adenovirus with fever versus adenovirus without fever). One program considered the best profile to be the one with the minimum number of classifiers. “With 18 classifiers, we got very nice discrimination between bacterial and viral cases,” Dr. Storch says. In this pattern, dysregulation was seen in 16 viral response genes and two bacterial response genes (the number of genes dysregulated in each type of infection).
Next, he says, “We tried to outsmart the program.” They created a “balanced” profile—one containing about the same number of viral and bacterial response genes. Finally, they put together their “best” panel, which turned out to contain 33 genes. The minimum number of classifiers remained 18. Most important, the ability to create such profiles proved hypothesis No. 1: Profiles can distinguish between viral and bacterial infections.
Hypothesis No. 2 also was proved. For instance, the profile of symptomatic HHV-6 infection could be distinguished from that of afebrile controls. Moreover, the profile of HHV afebrile “infection” was like that of afebrile controls. In general, profiles in children in whom virus was detected but no fever was present were identical to those in children with no virus. “This is intuitively right,” Dr. Storch says. “The host is not responding to that virus.”
How can these findings be translated for the clinical laboratory?
“It is definitely true that the microarray is not a clinically relevant technique, especially for infectious diseases,” Dr. Storch says. However, a multiplex real-time PCR assay interrogating the expression of 18 human genes could be useful for the clinical laboratory. Another possible approach: Look at the proteins that the altered genes encode. “These proteins could serve as biomarkers,” he says.
Dr. Christopher Woods, of Duke, became interested in harnessing the host response using genomics in early 2000. He was an infectious diseases fellow at Duke at the time and had been collaborating with David Relman, MD, of Stanford University. “We thought it would be great to use some of the approaches in early cancer diagnosis, especially microarrays, to help categorize infectious diseases,” he says.
At that time, Dr. Woods was going to Nepal to set up a large fever study. “What I talked David into doing with me was to look at host gene expression, which was a new tool at that time,” Dr. Woods says. However, when the data came back, “we really didn’t have the analytical statistical tools. Over the next several years, we made great leaps in our ability to work with these large databases, and we are also now better at pathogen detection.”
Dr. Woods subsequently joined the faculty at Duke and in the mid-2000s began collaborating with Geoffrey S. Ginsburg, MD, PhD, who is now a professor of medicine and pathology and director of the Center for Genomic Medicine at Duke. Their group got a large grant through DARPA (Defense Advanced Research Projects Agency) in the “Predicting Health and Disease” program. For the military, predicting whether a group of soldiers will become sick can influence the decision to deploy.
“We did numerous human biological challenges to see how you can detect when people become infected over time,” Dr. Woods says. This work couldn’t be done in mice. Fortunately, challenges with human rhinovirus and influenza virus had been done safely for many years, particularly in the United Kingdom. The Duke group derived a pan-respiratory viral diagnostic signature, including rhinovirus, respiratory syncytial virus, and influenza. “We could tell when someone was sick with a viral infection,” Dr. Woods says. “And we could push back in time to predict that they were going to become sick.” About 30 genes are involved in the original viral signature.
To validate the signature, they set up observational cohort studies on the Duke campus for several consecutive years. Students completed daily surveys. When students became sick, close contacts—people likely to develop symptoms—were enrolled. “In this work we validated the presymptomatic component of the signature,” Dr. Woods says. “We also validated symptomatic signature in emergency department populations. Now we can detect people who are sick with a virus and those who will become sick, before they show symptoms.”
The signature was validated to have about 90 percent accuracy in identifying infections due to respiratory viruses and in distinguishing them from bacterial respiratory infections (Zaas AK, et al. Cell Host Microbe. 2009;6:207–217). “I would argue that the potential for misclassification is as great with traditional diagnostics as with our approach,” he says. The expression profile complements traditional pathology-based approaches. “It certainly won’t replace them,” he says. An expression profile would give a clinician a basis for action while awaiting results of traditional tests.
In work led by group member Aimee K. Zaas, MD, MHS, associate professor of medicine at Duke, an RT-PCR-based assay for the gene signature was developed and validated (Zaas AK, et al. Sci Transl Med. 2013;5:203ra126). “This work has paved the way for a true clinical test,” Dr. Woods says.
“Now we need to ask, How do you make a difference? If you give a viral signature to clinicians, is that sufficient for them to withhold antibiotics?” In conversations with physicians in the ED, in critical care and in other clinical situations, the group found that many wanted a bacterial signature as well. “There is always the possibility for co-infections,” Dr. Woods says. “A viral infection may set you up for a bacterial infection.”
Since human bacterial challenge models are not established, they spent a lot of time developing a bacterial signature in ED cohorts where people present with confirmed bacterial infections. This work has not yet been published. “We are now finalizing the signatures and will put them together on a single platform to help people distinguish bacterial from viral from noninfectious respiratory illness,” Dr. Woods says. Results from the test could be available to clinicians in as little as two hours.
One of the greatest limitations of this approach, he explains, is that it will probably not be possible to distinguish infections due to various types of bacteria or to identify resistance. “We may be able to get influenza versus noninfluenza,” he says, “but we are not likely to be able to break it down into all virus groups.”
However, the generic nature of the signatures can be a strength in pandemics. “When the next MERS or SARS happens,” Dr. Woods says, “we can assume that the host response will be similar to what was generated with known influenza and respiratory viruses. One can imagine stockpiling diagnostic tools to identify an outbreak, due to the agnostic nature of this approach to pathogens.”
Deep sequencing without any preconceived specificity toward a certain pathogen makes it possible to look for new pathogens, ARUP’s Dr. David Hillyard says of next-generation sequencing. “As one does that, we continue to discover new pathogens and new variations on old pathogens.” But the heart of the work done at Duke and Washington University, he says, is not necessarily that we expect to be frequently surprised by new pathogens causing human disease. “Rather, what is the potential for understanding the signals that are coming from the host when there is an infection by a potential pathogen that actually causes disease? And what that could mean if it were packaged as a rapid, affordable test to aid clinical decisionmaking.”
There are many examples of people carrying bacteria and viruses that do not lead to serious disease, he says. “We want to bring to bear antibiotics and other interventions for people who have really serious disease.”
The discovery pathways of Duke and Washington universities are impractical for clinical testing, he says, and different from the kind of quantitative transcript testing that would likely be part of a commonly used clinical laboratory assay. “In both cases, an essential task is to reduce the number of candidate transcripts to a smaller number that can be part of a practical assay,” Dr. Hillyard says.
Dr. Christine Ginocchio, of Hofstra, raises another problem: the need for a sufficiently high concentration of pathogen nucleic acid to allow direct detection by PCR from blood. “There are new diagnostic methods that incorporate steps to accomplish this but still need to be refined to provide sufficient sensitivity,” she says. “We can do it now with many viral pathogens as the concentration in the blood is relatively high, but not yet with sufficient sensitivity for many bacterial pathogens.” Within the next couple of years, she predicts, “we will be able to move toward direct detection.”
Dr. Ginocchio agrees that working with the shotgun approach of NGS using very broad-based primers is a great way to discover new viruses. She also agrees that from a diagnostic standpoint at this time, it would be difficult for clinical laboratories to do. “It is getting easier, but the up-front sample processing steps and the informatics part of NGS are still very difficult for the routine laboratory to handle.”
Dr. Ginocchio sees NGS as being where mass spectrometry was 10 years ago.
“Now MALDI-TOF mass spectrometry is a black box—sample in, result out,” she says. “Eventually, deep sequencing for diagnostic applications will need to be as simple for acceptance as a routine test method.” It will become more prevalent in clinical diagnostics laboratories in, she hopes, the next five years. “Deep sequencing will be important for samples where there might be a broad range of pathogens and for when we do not always know what pathogen we are looking for, such as joint fluids.” Though it may not be for every clinical laboratory, it will be a tool used in reference and university labs within the near future. “A lot of large laboratories already do next-gen sequencing for genetic applications, such as testing for inherited diseases. It is definitely coming to the infectious disease diagnostics side and will also be a powerful epidemiology tool,” she says.
In many situations, though, such as for patients seen in the emergency department, NGS will not be fast enough. “We often want an answer in an hour. We can do broad-range PCR for the top 20 or 30 bacteria in a few hours,” she says.
Gene expression profiles will also need to have a turnaround time of about one hour, Dr. Ginocchio says, at least for critical decisionmaking for ED and ICU patients. “Now [the researchers] have to go into the translational medicine stage and take the biomarkers they have identified and put them into an assay that is fast and simple enough for the clinical laboratory to run. All of that is doable,” Dr. Ginocchio says. And, indeed, it is already being done.
Even when expression profiles are translated into a usable diagnostic format, one more possible obstacle will remain: getting clinicians to adopt the new technology. “Oftentimes, for diagnostics in particular,” Dr. Woods says, “clinicians tend to be slow adopters for doing things that maybe many of them don’t understand. It is incumbent on us to educate and teach people to use this new tool.”
Dr. Storch agrees, and he stresses the power of replication: “The more we can replicate one another’s results, the better it will be.” This is particularly true with regard to informatics, Dr. Storch says. “It feels good when you have multiple statistical groups working with the data and hopefully generating the same result using different approaches. That’s an important part of the process.”
Dr. Hillyard calls the work by the Duke and Washington University groups “pioneering research projects.”
“The whole world [of microbiology] is watching,” he says. “What remains is for these investigators to demonstrate sensitivity and specificity for these unique subsets of transcripts.”
[hr]
William Check is a writer in Ft. Lauderdale, Fla.