September 2023—New from CAP Publications is Disruptive Technologies in Clinical Medicine, by Frederick Kiechle, MD, PhD. In his new book Dr. Kiechle says “disruptive technologies offer new paradigms in diagnostic medicine.” Technology-driven disruptions are stimulated by the need to improve patient care, he writes, and they have been “a feature of the practice of clinical pathology since the inception of the first clinical laboratory in 1895 at the University of Pennsylvania, the William Pepper Laboratory.”
It was at the University of Pennsylvania that Dr. Kiechle began his clinical pathology career in 1980. He moved to William Beaumont Hospital in Michigan in 1983 and then to Pathology Consultants of South Broward in Florida in 2006. Since 2018 he has been chief medical officer of Boca Biolistics Reference Laboratory in Florida.
CAP TODAY asked Dr. Kiechle about his new book; his answers follow. Part of his chapter on phlebotomy begins below.
You write in the book about biosensors, microfluidics, artificial intelligence, CRISPR, and many other technologies. Tell us about your topics and how you selected them.
The book reviews disruptive technologies and events that have been selected based on their past, present, or future impact on workflow in clinical medicine or clinical pathology practice, or both. These topics include three event-driven disruptive technologies: infectious disease, personal behavior, and cultural norms—cis/trans. Also reviewed are 12 technical disruptive technologies: biosensors for physiological monitoring and biochemical sensors, smartphones, MALDI-TOF MS, microbiome, phlebotomy practice, point-of-care testing, microfluidics, molecular diagnosis (oncology, single molecule sequencing, isothermal amplification, CRISPR/retrons, pharmacogenomics), organoids, organ-on-a-chip, and artificial intelligence.
There’s a table in the book that lists the disruptive technologies you experienced in your own career. It includes molecular diagnostics in 1993 when you initiated, with Dan Farkas, PhD, one of the first molecular diagnostic labs in the U.S.; introducing MALDI-TOF in microbiology in 2010 with Rodney Arcenas, PhD; and establishing SARS-CoV-2 molecular and immunoassay testing in 2020 with Gloria Shore, MT(ASCP), of Boca Biolistics and Boca’s CEO Val Aida and COO Michael Morris, to name just a few technologies on the list. Is there one disruptive technology that stands out in your career as most disruptive, most difficult, most interesting, or having provided the greatest number of lessons learned?

Dr. Kiechle
The introduction of point-of-care testing at alternative sites, which redistributed laboratory testing like glucose much closer to the patient’s bedside, was probably the most disruptive, difficult technology. Blood glucose analysis has for many years been performed in the centralized clinical chemistry laboratory. Patients and endocrinologists required a more rapid turnaround time, especially when the central lab glucose turnaround time was 60 minutes and the patient’s insulin drip needed to be adjusted every 30 minutes. Clinical biosensors were developed to measure plasma glucose from a fingerstick and later small needles that penetrate the skin into the interstitial space to measure the interstitial fluid glucose.
Clinical laboratorians initially had numerous concerns about the decentralization of glucose testing, but many of the issues were resolved after the development of various guidelines for establishing a decentralized hospital or non-hospital–based location. This approach to decentralized testing is now being used for a variety of analytes like intraoperative PTH, drug screening, and viral and malarial detection. Lateral flow methods have been used for these commercial products as well.
You’re well known for your expertise in phlebotomy and the CAP manual So You’re Going to Collect a Blood Specimen. There’s a chapter on phlebotomy in your new book. What do you foresee phlebotomy practice to be like 10 years from now?
It is always difficult to anticipate what new disruptive technology will be introduced to improve phlebotomy practice. There are methods available now for visualizing veins close to the skin and ultrasound-guided peripheral intravenous cannulation, which I write about in chapter five. In the next 10 years, I would anticipate the introduction of several needleless phlebotomy devices. Non-penetrable biosensors using osmotic techniques like current sweat chloride collection may be used. In the future, biosensors placed directly on the skin will be used to measure all the chemistry tests in a comprehensive metabolic panel and others.
In your chapter on AI, there’s a table on examples of AI models in clinical pathology, two of which are personalized antibiograms and plasma amino acid interpretation. Can you elaborate on one or both of those examples or any other example on the list?
Chapter 10 is devoted to artificial intelligence applications in clinical medicine, anatomic pathology, and clinical pathology. This chapter was completed before chatbot was introduced. Chatbot is a computer program designed to simulate conversation usually over the internet. There are now at least six types of chatbots: menu/button based, linguistic based (rule based), keyword recognition based, machine learning, hybrid model, or voice bots.
One example of AI models in clinical pathology is its use in the complex interpretation of plasma among acids used to diagnose and monitor inherited disorders of amino acid synthesis, catabolism, and transport, as well as organic acidemias and urea cycle defects (Wilkes EH, et al. Clin Chem. 2020;66[9]:1210–1218). Interpretation of these amino acid profiles can’t rely on differences in the actual concentration of the amino acid detected by liquid chromatography–tandem mass spectrometry. An assessment of the profile as a whole is required to distinguish the 50 different disorders diagnosed from a plasma amino acid profile.
Table 45 in chapter 10—examples of AI models in clinical pathology—lists the supervision type and algorithms used in the Clinical Chemistry study. Supervision type defines the amount of labeled and/or unlabeled data that’s used. In supervised learning a supervisor is used to teach or train the machine using well-labeled data. Semi-supervised refers to a mix of labeled and unlabeled data. An unsupervised machine learning algorithm uses methods to analyze and cluster unlabeled data sets. The plasma acid interpretation study used supervised learning followed by the comparison of interpretation accuracy using three machine learning algorithms: random forest nonlinear, weighted-subspace random forests, and extreme gradient boosted trees (XG Boost).
Briefly, random forest nonlinear uses multiple decision trees for predicting the output compared with only one tree used in decision tree regression. Weighted-subspace random forest was introduced in 2012 to classify high-dimensional data with random forests built using small subspaces. XG Boost is a scalable boosted decision tree machine learning library. It provides parallel tree boosting for regression, classification, and ranking problems. Common biases like race, ethnicity, and gender may be hidden in the labeled or unlabeled data sets. These biases will then be transferred to the machine learning algorithm. These issues need to be understood and detected early to avoid interpretation bias. In the plasma amino acid interpretation of three machine learning algorithms, all three provided excellent predictive performances. Application of AI to clinical medicine and/or clinical laboratory application is in its infancy of development.
Why did you decide to write the book and what are you hoping the reader will take away from it?
I was stimulated to write this overview of disruptive technologies and events to assess the issues faced as a clinical pathologist laboratory director for more than 30 years. It also provided an opportunity to evaluate the historical growth development and impact of past, present, and future disruptive technologies and events. The major takeaway from reading this overview is to develop a sense of how these pervasive disruptive developments will influence your own clinical pathology laboratory workflow, which may require change in personnel skills and workstation development.
Below, from Disruptive Technologies in Clinical Medicine by Frederick Kiechle, MD, PhD, is part of chapter five on phlebotomy, with only some of what Dr. Kiechle wrote on the topic of lateral flow methods (and without the chapter references). To order (PUB318), call 800-323-4040 option 1 or go to www.cap.org (Shop tab) ($36 for members, $45 for others; ebook: $32). If you are interested in writing a book, contact Katy Meyer at kmeyer@cap.org.
Phlebotomy
Phlebotomy is the practice of drawing blood for laboratory patients. The location of the vein in the patient by manual palpation may be difficult. Alternative methods, transillumination, or ultrasound guidance may aid in this process.
A. Aids for Visualizing Veins Under the Skin
Good visualization of veins in the arm or back of the hand using a fiberoptic light source will increase the success rate of venipuncture in pediatric patients to 100% from 83% without the aid of transmitted light. The height or weight of a patient does not predict the rate of successful phlebotomy. Current strategies to improve the visualization of veins include glasses with special lenses that include filters that enhance the exact color of deoxygenated blood under the skin and enhance the location of veins and near-infrared (740-760 nm) light source illumination and orange/red LED side transillumination. These systems visualize veins situated 3-5 mm under the skin. A comparison of three near-infrared skin illumination devices (VeinViewer, ICEN IN-G090-2, AccuVein AV400) demonstrates that these devices have different image visibility under different viewing angles, lighting, humidity interference and presence of body lotions. Veinsite and Penlite LP212 had shorter times to successful venipuncture compared to controls (tourniquet). The effect of tourniquet time vs infrared illumination without a tourniquet revealed that there are measurement errors after 60 seconds of tourniquet time.
Near-Infrared Light Illumination
- VeinViewer. Christie Medical Holdings, Memphis, TN – www.christiemed.com
- AccuVein. AccuVein Inc, Cold Spring Harbor, NY – www.accuvein.com
- VascuLuminator. De Koningh Medical Products, Arnhem, NL – www.do-pa.com
- Veinsite system, including head mount, portable near-infrared emitter, video acquisition and display device. VueTek Scientific, Gray, ME – www.cience.com/company/vuetek-scientific/3492254564897256528
- Eyes-On-Glasses. Evena Medical, Los Altos, CA – www.evenamed.com
- VenoscÓpio IV. Duan Internacional do Brasil, Brazil – www.duaninternacional.com
- Penlite LP212. Energizer, St. Louis, Missouri – www.energizerholdings.com
- IN-G090-2. ICEN Technology Company Limited, Guangdong, China – www.icenteco.com
B. Ultrasound-Guided Peripheral Intravenous Cannulation
Patients who are obese, diabetic, have chronic kidney disease, abuse drugs, or have had numerous intravenous catheters may present with peripheral difficult intravenous access (DIVA). Ultrasound guidance has higher success rates for veins with a diameter >0.4 cm compared to <0.4 cm and with veins at a depth of 0.3 to 1.5 cm compared to <0.3 or >1.5 cm. In contrast, near-infrared visualized veins are only 3 to 5 mm under the skin (Chapter 5A). A prototype image-guided autonomous venipuncture robot has been reported that used both near-infrared and ultrasound guidance systems to visualize and puncture a vein. Some hospitals have formed vascular access teams that specialize in performing ultrasound-guided venipuncture in DIVA patients.
During ultrasound guidance, a gel is required to ensure acoustic coupling between the skin and the transducer. This gel will be penetrated by the needle and may lead to complications, like infection. A barrier drape, which consists of a transparent piece of sterile plastic, has been described that separates the gel and transducer from a dry skin area used for the venipuncture. Ultrasound images show dark vascular fluid-filled veins or arteries and light surrounding tissues.
The transverse view is the easier technique, illustrating cross-sections of vessels. Veins are compressible with light transducer pressure and can be differentiated from arteries by the absence of visible pulsation. The longitudinal technique is more difficult and illustrates a vein or artery as a tubular structure. Ultrasound-guided venipuncture is the preferred method for central venous catheter placement.
C. Needleless Venipuncture, Sample Types and Lateral Flow Methods
There has been a major effort to reduce needlestick injuries, which represent 13 to 62% of injuries reported to the Hospital Occupational Health Service. Ballout, et al reported moderate quality evidence that phlebotomy safety devices reduce the risk of needlestick injuries in healthcare workers, but have no effect on bloodborne pathogen infections. Butterfly needles are more commonly used for venipuncture than a needle and syringe or indwelling IV catheter. In an emergency department, butterfly phlebotomy reduced the incidence of hemolysis by more than half compared to IV catheter phlebotomy. Arm venipuncture is usually performed in the antecubital fossa using the preferred median cubital vein rather than the cephalic vein which tends to roll. Never use the basilic vein since it is close to the brachial nerve.
Disposable, automated, retractable lancet devices have been introduced. For fingerstick collection, the proper size lancet device is required. For adults, the puncture depth should be between 2.2 to 2.5 mm. For children greater than 20 lbs, 1.7 to 2.0 mm puncture depth is recommended. Table 1 reviews the appropriate depth for lancet penetration for infants <1 kg (2.2 lbs) to 3 kg (6.6 lbs) or greater. In general, blood volume collected increases with the lancet diameter and penetration depth. Lancet devices with triggered-linked lancet movement were significantly less painful than capillary fingersticks. Capillary fingersticks should puncture the side of the finger pad cutting across the lines of the finger and not along the lines of the finger. For neonatal heelsticks, the back sides of the heel should be used. First, prewarm the infant’s heel (no higher than 42º C) for 3 to 5 minutes. Use Table 1 to select the appropriate retractable lancet size. The order of drawing specimens differs for capillary blood draws compared to venipuncture (Table 2).
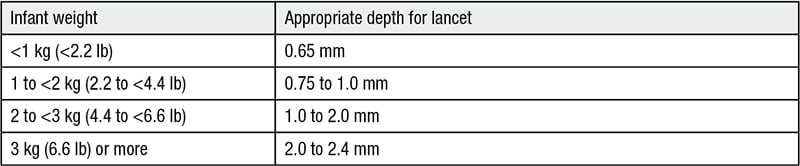
Table 1. Appropriate retractable lancet size for heelsticks in infants
• Use a sterile lancet to puncture the side of the heel. To avoid injury to underlying bone, which is close to the skin surface, do not puncture the central portion of the heel.
• Never twist the lancet to make a larger hole to enhance bleeding.
The ultimate goal is to develop a needle-free venipuncture technique. The Defense Threat Reduction Agency (DTRA) Chemical and Biological Technologies Department is supporting the modification of an existing commercially available push button device to increase the volume collected from 200 μL to 750 μL. The current commercial device attaches to the patient’s skin by an adhesive ring. It draws blood after a button is pushed by activating lancets or needles to gently puncture the skin and capture blood in a self-sealing vacuum tube. The device is easy to use and less painful than a finger prick. It is designed for use on the battlefield.
Another approach, called PIVO (Velano Vascular, San Francisco, CA), used a polymer catheter that is threaded through the existing short peripheral catheter past the hub and end of the short peripheral catheter tip into the vein. PIVO received FDA clearance in 2015. Studies comparing PIVO blood specimens with blood drawn directly from peripheral venous catheters showed significant difference in hemolysis on lab test results. After completion of blood collection, the PIVO polymer catheter is retracted and removed from the IV and discarded. PIVO requires that the patients have a short peripheral catheter in place prior to its use. This would increase the use of peripheral catheter, but would markedly reduce the 10 to 20 needlesticks each of the 400 million inpatients receive per hospital stay. The goal of needleless venipuncture is to reduce the pain of repeated venipuncture, needlestick injuries and potential associated bloodborne infection like HBV, HCV, HIV, etc.
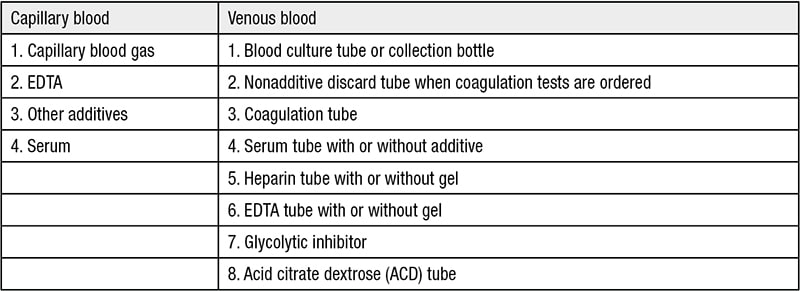
Table 2. Order of draw
Blood specimen types include whole blood, plasma and serum. Blood contains liquid components and cellular components. When anticoagulant is added to prevent clotting, the liquid component of blood is called plasma. When blood is allowed to clot in a tube, the resulting liquid is called serum. Serum does not contain all the proteins present in plasma because some proteins, clotting factors, are consumed when blood clots. There are four anticoagulants that can be added to the collection tube to prevent clotting: EDTA, sodium citrate, heparin and fluoride-oxalate.
Another approach to reduce the frequency and amount of blood collected is to reduce the volume of plasma/serum needed for analysis using microfluidics and/or lateral flow analysis. The Velvet system (Weavr Health, Cambridge, MA) uses lithium heparin whole blood (180-240 μL) added to a collection device and separates the cellular components from the plasma after drying the device over 12 hours. The dried spots (cellular and plasma) are removed from the paper lateral flow device using a punch. These punches are rehydrated in diluent and analyzed using routine laboratory equipment. A pilot project successfully provided accurate measurements for hemoglobin A1C in the cellular component from the paper lateral flow system and cholesterol, HDL, LDL, triglycerides and chloride from the plasma. Chloride was used to normalize variability secondary to extraction and undefined plasma volume. A blood collection device using blood-based lateral flow to filter out red blood cells without hemolysis (DCNovations, DCN Dx, Carlsbad, CA) provides plasma to be used for analyte testing.